INTRODUCTION
Trisomy 18 (T18) and trisomy 21 (T21) are caused by chromosomal abnormalities, leading to intellectual disabilities and various health complications ( Buczyńska et al., 2022). The chromosomal abnormalities in the T18 and T21 gene regions cause a wide variety of health problems including developmental impairments ( Bihunyak et al., 2020). Both conditions involve the presence of an extra copy of chromosome 18 or 21, respectively, in some or all of the body’s cells ( Levy et al., 2021). These chromosomal abnormalities occur during the fertilization process or the early stages of development ( Viotti, 2020). T18 and T21 are associated with intellectual disabilities. Individuals with these conditions may have difficulty in learning and may face challenges with language skills, problem-solving abilities, and adaptive functioning ( Bukaty, 2016). These disabilities can range from mild to moderate, depending on the specific case and the severity of any additional health complications. In addition to intellectual disabilities, individuals with T18 and T21 often experience various health complications ( Eldeniz Çetin and Çay, 2020). These can include heart defects, respiratory problems, feeding difficulties, developmental delays, and seizures. Some individuals may also have kidney abnormalities, gastrointestinal issues, and abnormalities in the central nervous system ( Zheng et al., 2022).
Individuals with T18 and T21 are at an increased risk of developing other health conditions. These can include congenital anomalies such as cleft lip and palate, as well as various genetic syndromes such as Down syndrome and Fragile X syndrome ( Verma, 2021). Additionally, individuals with these conditions may be more susceptible to infections and have a higher likelihood of complications during pregnancy. Living with T18 and T21 can be challenging for both individuals and families ( Bal et al., 2015). Support and resources are available to assist, including early intervention programs, special education services, and support groups. Additionally, healthcare professionals can provide guidance and support in managing the various health complications associated with these conditions ( Bruns and Foerster, 2011). Molecular markers are specific genetic or biochemical changes in an organism’s body that serve as indicators of a particular condition or disease. Advances in our understanding of molecular markers have enabled the identification of novel biomarkers for prenatal screening ( Pös et al., 2019).
TRADITIONAL SCREENING METHODS
One of the key diagnostic tools used for diagnosing both T18 and T21 is the identification of biochemical markers ( Norwitz and Levy, 2013). Biochemical markers are substances that can be measured in the blood or other substances to provide information about the presence or absence of a specific condition ( Goldenberg et al., 2005).
Biochemical markers that are commonly used in the diagnosis of T18
Ketogenic acid levels
Increased levels of ketones, which are acidic by-products of fat breakdown, can be detected in the blood of individuals affected by T18. However, it is important to note that this marker is not specific to T18 and can also be present in other conditions such as diabetic ketoacidosis ( McTaggart et al., 2021).
Elevated immunoreactive trypsinogen
Increased levels of immunoreactive trypsinogen, an enzyme involved in the digestion process, have been observed in the blood of T18 patients ( Alghamdi, 2011).
Elevated alpha-fetoprotein levels
Alpha-fetoprotein (AFP) is a protein produced by the liver and released into the bloodstream. Elevated levels of AFP, particularly in the early stages of pregnancy, may indicate an increased risk of T18 ( Yoshizato et al., 2019).
Elevated levels of human chorionic gonadotropin
Human chorionic gonadotropin (hCG) is a hormone produced during pregnancy. Elevated levels of hCG, particularly in the early stages of pregnancy, may indicate an increased risk of T21 ( Atallah et al., 2023).
Unconjugated estriol
Lower levels of unconjugated estriol have been associated with T21 pregnancies. Estriol is a form of estrogen produced by both the fetus and the placenta.
Nuchal translucency
Nuchal translucency testing is used in conjunction with other screening tests, such as first-trimester screening or combined first-trimester screening, to identify potential chromosomal abnormalities ( Nicolaides, 2004). It is particularly valuable in cases of T18 and T21 because these conditions often have certain physical characteristics that can be detected by ultrasound. Nuchal translucency is measured using an ultrasound device called a sonogram ( Nicolaides, 2004). The sonographer will position the device over the neck of the developing fetus and measure the thickness of the translucent area behind the neck. This measurement, known as the nuchal translucency thickness, is then compared to established reference ranges to identify any abnormalities ( Nicolaides, 2004). Nuchal translucency thickness can vary during pregnancy, and the normal range can vary depending on factors such as the gestational age and gender of the baby ( Nicolaides et al., 2002). However, an elevated nuchal translucency thickness can indicate a higher risk for T18 or T21 ( Kagan et al., 2006).
Nuchal translucency results in conjunction with other screening tests, such as maternal age, blood test results, and medical history, can help healthcare providers evaluate the risk of T18 or T21. An elevated nuchal translucency thickness may be further investigated with diagnostic tests, such as amniocentesis or chorionic villus sampling (CVS), to confirm the diagnosis and provide more precise information ( Kelley et al., 2021).
EMERGING BIOMARKERS FOR PRENATAL SCREENING
Prenatal screening for these conditions has evolved, with increasing emphasis on identifying specific biomarkers for accurate and early detection ( Krstić and Običan, 2020). Prenatal screening for T18 and T21 is crucial for identifying the risk of these conditions during pregnancy ( Rose et al., 2022). In recent years, advancements in genomic technologies and our understanding of molecular markers have revolutionized the field, paving the way for the development of novel biomarkers that can enhance the precision and reliability of prenatal screening ( Fig. 1) ( Raghavendra and Pullaiah, 2018).
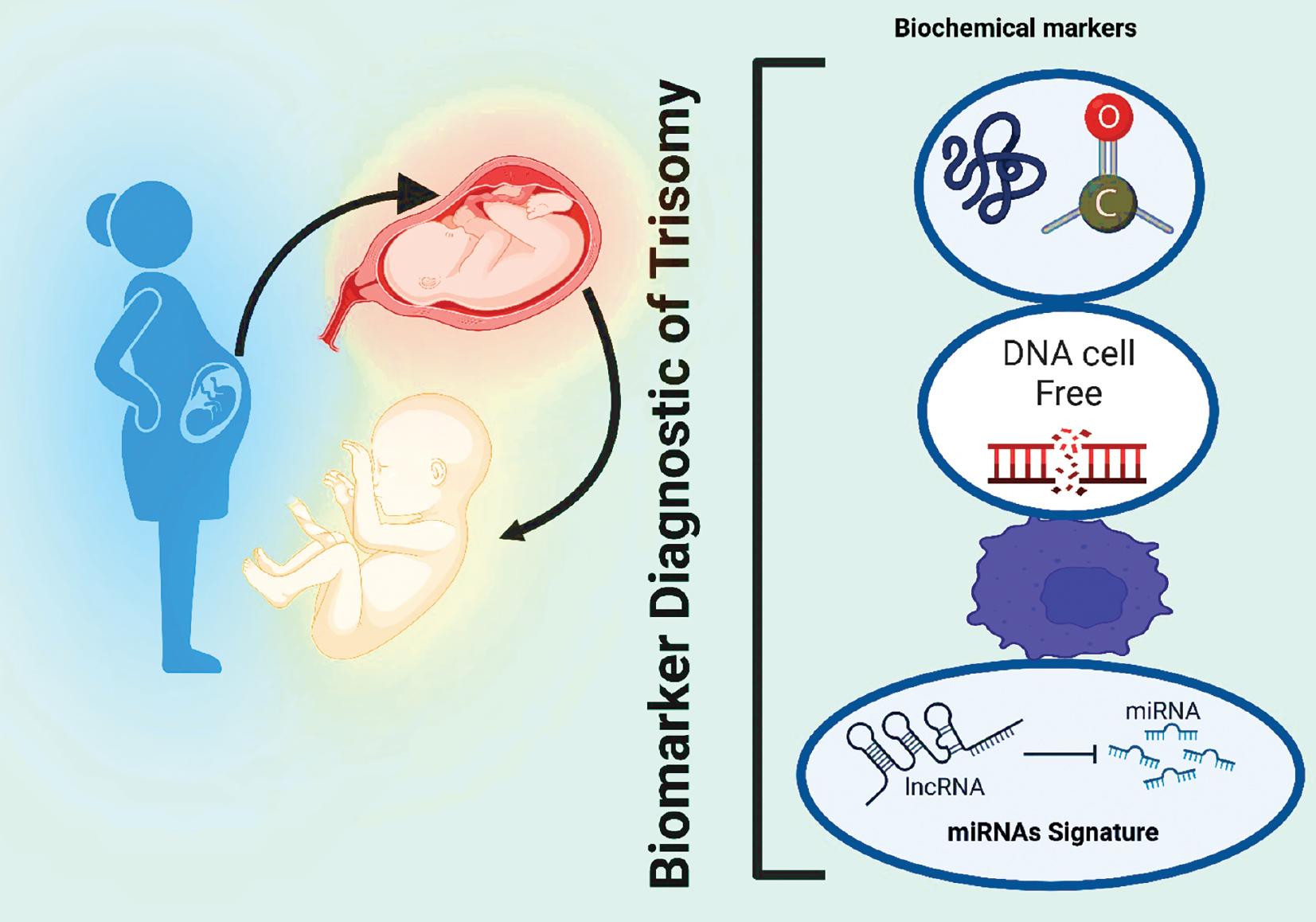
Screening for prenatal defects using emerging biomarkers. Prenatal screening can help identify potential genetic or chromosomal abnormalities in the fetus before birth, allowing parents to make informed decisions about the care of their baby. By screening for these biomarkers, doctors can identify potential problems before the baby is born and provide appropriate treatment if necessary. Abbreviations: lncRNA, long noncoding RNA; miRNA, microRNA.
Noninvasive prenatal testing
Noninvasive prenatal testing (NIPT) has gained significant attention in recent years as a reliable and accurate method for prenatal screening. NIPT involves analyzing cell-free fetal DNA obtained from a mother’s blood sample. By examining the DNA for genetic abnormalities, NIPT can provide an early and accurate risk assessment for T18 and T21 ( Cai et al., 2023).
Cell-free DNA
Landscape of cfDNA testing
Cell-free DNA (cfDNA) has gained significant attraction due to its noninvasive nature and higher accuracy compared to conventional methods. It involves analyzing cfDNA in the mother’s bloodstream to detect the presence of specific genetic abnormalities ( Song et al., 2022). The test can be performed as early as the 10th week of pregnancy, providing expectant parents with timely information ( Balaguer et al., 2024). Currently, there are three main methods of cfDNA testing: quantitative polymerase chain reaction (qPCR), sequencing-based tests, and microarray-based tests. Each method has its advantages and limitations, but in general, cfDNA testing has shown promising results in detecting T18 and T21 ( Pérez-Barrios et al., 2016). One of the novel biomarkers that have been explored for the prenatal screening of T18 and T21 is fetal DNA in maternal blood. Fetal DNA can be found in the mother’s bloodstream during pregnancy, and by analyzing this DNA, it is possible to detect genetic abnormalities in the developing fetus. This technique has shown promise in improving the accuracy and reliability of prenatal screening for T18 and T21 ( Shi et al., 2019).
Accuracy of cfDNA testing
The accuracy of cfDNA testing for detecting T18 and T21 has been widely studied and demonstrated ( Wang et al., 2021). Several studies have reported sensitivities and specificities ranging from 95% to 100% ( Yu et al., 2021; Raymond et al., 2023). This means that, for the vast majority of cases, the test accurately identifies pregnancies affected by these conditions. The high accuracy of cfDNA testing can be attributed to several factors. First, cfDNA is readily available in the maternal bloodstream, allowing for easy sampling and detection. Second, the methodology used in cfDNA testing, such as qPCR and sequencing, allows for precise analysis and quantification of specific genetic markers associated with T18 and T21 ( Shaw et al., 2020).
Challenges and false positives and negatives
While cfDNA testing has revolutionized prenatal care, it is not without limitations. One significant challenge lies in distinguishing between a true-positive result, indicating the presence of a genetic condition, and a false-positive result, indicating a falsely elevated risk. False positives can occur due to various factors such as maternal contamination, incidental fetal mosaicism, or laboratory errors ( Grati, 2014). On the other hand, false negatives can also occur with cfDNA testing. These false negatives occur when the test fails to correctly identify pregnancies affected by T18 or T21. This can occur due to limitations in the test’s ability to detect certain genetic abnormalities or due to underlying factors that interfere with the test’s accuracy ( Mennuti et al., 2013).
MicroRNA signatures
In recent years, microRNAs (miRNAs) have emerged as promising candidates for biomarkers in prenatal screening. miRNAs are small noncoding RNA molecules that regulate gene expression and play a crucial role in various cellular processes. Studies have shown that dysregulated miRNA expression patterns in the placenta and amniotic fluid associated with T18 and T21 can serve as biomarkers for prenatal diagnosis ( Chavira-Suárez et al., 2021; Seelan et al., 2022).
Promise of miRNA-based screening for T18 and T21
MiRNA-based screening offers several advantages over traditional diagnostic methods. First, miRNAs are stable in biological fluids, such as amniotic fluid or maternal serum, making them easily accessible for analysis ( Mestry et al., 2023). Second, miRNAs are highly specific and can detect the presence of specific chromosomal abnormalities, reducing the chances of false positives or negatives. Furthermore, miRNAs can serve as early biomarkers, providing valuable information before the development of visible signs or symptoms ( Yang et al., 2020). This could potentially enable earlier intervention and personalized treatment plans for affected individuals. Moreover, miRNAs offer the possibility of noninvasive screening methods, eliminating the risks associated with traditional amniocentesis ( Vandepitte, 2022).
CHALLENGES OF miRNA-BASED SCREENING FOR T18 AND T21
While the promise of miRNA-based screening for T18 and T21 is exciting, several challenges need to be addressed. First, the identification of reliable miRNA biomarkers remains an ongoing area of research. The complexity of miRNA expression patterns in the placenta and amniotic fluid makes it difficult to identify specific miRNAs that can accurately distinguish T18 and T21 from other chromosomal abnormalities ( Olaya Agudo, 2015). Additionally, the interpretation of miRNA data can be challenging. The regulation of gene expression by miRNAs involves complex mechanisms, and variations in miRNA expression can occur due to environmental factors or normal developmental processes ( Gulyaeva and Kushlinskiy, 2016). These factors need to be taken into account when assessing the significance of miRNA expression patterns for prenatal diagnosis. Furthermore, the standardization and validation of miRNA-based screening methods need to be established to ensure accurate and reliable results. Standard operating procedures, quality control measures, and proficiency testing programs are essential to ensure consistent and accurate diagnosis across different laboratories ( Precazzini et al., 2021).
PROTEOMIC AND METABOLOMIC APPROACHES
Trisomy refers to the presence of an extra copy of a chromosome, which can significantly disrupt normal embryonic development and often lead to severe health complications. Traditional screening methods, such as ultrasound, amniocentesis, and CVS, have played a crucial role in identifying trisomy pregnancies, but their accuracy is limited. In recent years, proteomic and metabolomic approaches have emerged as powerful tools for detecting trisomy, offering complementary perspectives to traditional screening methods ( Monni et al., 2021).
Proteomic profiles
Proteomic analysis involves the systematic identification and quantification of proteins in a biological sample ( Brewis and Brennan, 2010). By analyzing protein abundance, researchers can gain insights into the functional and metabolic changes associated with trisomy. Several studies have utilized proteomics to identify specific protein markers that differentiate trisomy pregnancies from normal pregnancies ( Vasani and Kumar, 2019; Gao et al., 2021). One such study utilized mass spectrometry-based proteomics to identify differentially expressed proteins in the urine of pregnant women ( Al-Jasim, 2019). Several proteins, including retinol-binding protein 4, albumin, and keratin, were significantly altered in trisomy pregnancies, providing potential biomarkers for early detection ( Bujold et al., 2021).
Another study conducted by Bhatti et al. (2022) utilized a proteomic approach to analyze amniotic fluid from pregnant women. The study found that specific proteins, such as transthyretin and AFP, were altered in trisomy pregnancies, suggesting their potential as biomarkers for prenatal screening.
Metabolomic profiles
Metabolomics is the study of metabolites, the small molecules produced by cells. By analyzing the metabolomic profile of a biological sample, researchers can gain insights into the metabolic changes associated with trisomy. Metabolomics provides a holistic view of the metabolic landscape, offering unique insights into cellular processes and disease mechanisms ( Monni et al., 2021). Several studies have utilized metabolomics to investigate trisomy pregnancies ( Troisi et al., 2020; Dahabiyeh and Nimer, 2023). Nemutlu et al. (2019) used gas chromatography–mass spectrometry to analyze urine samples and found altered levels of metabolites associated with oxidative stress and lipid metabolism in trisomy pregnancies. These metabolites may provide valuable diagnostic clues for early detection. Another study by Naila et al. (2022) utilized nuclear magnetic resonance spectroscopy to analyze amniotic fluid from pregnant women. This study identified metabolite patterns that differed between trisomy pregnancies and normal pregnancies, suggesting the potential of metabolomics as a complementary approach for prenatal screening.
Potential as complementary approaches
While traditional screening methods remain essential for diagnosing trisomy pregnancies, proteomic and metabolomic approaches offer complementary perspectives ( Issaq and Veenstra, 2019). By identifying specific protein markers and altered metabolite profiles, these approaches can provide additional insights into the molecular changes associated with trisomy ( Buczyńska et al., 2021). The sensitivity, specificity, and noninvasive nature of proteomic and metabolomic techniques make them well suited for early screening. Additionally, these approaches have the potential to identify biomarkers that are missed by traditional screening methods ( Khoo et al., 2021).
GENOMIC TECHNOLOGIES FOR TRISOMY DETECTION
Trisomy detection is a crucial aspect of prenatal diagnosis, fetal medicine, and research. It involves identifying the presence of an extra copy of a chromosome in a fetus or embryo. Over the years, several genomic technologies have emerged to facilitate the accurate and reliable detection of trisomy ( Bedei et al., 2021). These technologies offer advantages over traditional methods, such as cytogenetic analysis, and have become essential tools in this field.
Microarray analysis
Microarray analysis is a revolutionary genomic technology that has revolutionized the field of trisomy detection ( Zhu et al., 2019). It involves the hybridization of fetal DNA to an array containing thousands of DNA probes representing different chromosomes ( Levy and Burnside, 2019). If an extra copy of a chromosome is present, it will result in a signal on the array, indicating the presence of the trisomy. Microarray analysis provides high-resolution genotyping, enabling the identification of small and mosaic trisomy cases. It also enables the simultaneous detection of multiple trisomy conditions, reducing the need for multiple invasive procedures ( Liu et al., 2022).
Next-generation sequencing
Next-generation sequencing (NGS) technologies, such as whole-exome sequencing and whole-genome sequencing, have also become powerful tools for trisomy detection ( Gabriel et al., 2022). NGS allows researchers to quickly sequence and analyze the entire genome or specific regions of the genome. By comparing the sequence of the fetus’s DNA to the reference genome, NGS can identify copy number variations, including trisomy ( Satam et al., 2023). NGS offers high sensitivity and specificity, allowing the detection of mosaic trisomy cases, which are challenging to detect using traditional methods ( Biricik et al., 2021). Genetic sequencing and analysis have emerged as powerful tools for understanding the human genome. These advancements have revolutionized the diagnosis of genetic disorders, including T21 and T18. With advancements in genetic sequencing technologies, such as NGS, it becomes possible to obtain a detailed genetic profile of the fetus. This genetic information can then be used to identify specific biomarkers that are associated with T21 and T18 ( Volk et al., 2013).
Fluorescent in situ hybridization
Fluorescent in situ hybridization (FISH) is another commonly used genomic technology for trisomy detection. It involves the use of fluorescently labeled DNA probes that bind to specific chromosomes ( Chrzanowska et al., 2020). By analyzing the pattern of fluorescence, researchers can detect the presence of an extra copy of a chromosome ( Freitag et al., 2021). FISH has certain limitations, such as the need for specialized expertise and the availability of specific probes for each chromosome. However, it remains a reliable and widely used technique for detecting trisomy ( Bhagat et al., 2020).
Polymerase chain reaction
Polymerase chain reaction (PCR) is a technique used to amplify specific DNA sequences. It has been employed for trisomy detection by amplifying specific regions of the chromosomes associated with trisomy conditions. PCR offers high sensitivity and accuracy, allowing the detection of small and mosaic trisomy cases. However, it requires specific primers and conditions for accurate amplification, and the results obtained with PCR alone must be confirmed using other genomic technologies ( Kim et al., 2021).
Genomic microarray comparative genomic hybridization
Genomic microarray comparative genomic hybridization (array CGH) combines the concepts of microarray analysis and CGH analysis. It compares the amount of DNA from the fetus’s chromosomes to a reference genome to identify copy number variations, including trisomy. Array CGH offers higher resolution and higher sensitivity compared to FISH, making it a valuable tool for trisomy detection. It also enables the simultaneous detection of multiple trisomy conditions in a single assay ( Wayhelova et al., 2019).
DIAGNOSTIC ACCURACY
The diagnostic accuracy of trisomy screening varies depending on the type of test used and the gestational age at which the test is performed ( Al Jashi and Al Jashi, 2019). In general, the risk of false-positive results is higher in early pregnancy, while the risk of false-negative results is higher in late pregnancy. False-positive results occur when the test suggests that a fetus has a genetic abnormality, but the abnormality is not present in reality. This can result in unnecessary anxiety and additional testing, as well as the potential termination of a pregnancy. On the other hand, false-negative results occur when the test fails to indicate that a fetus has a genetic abnormality, when in fact it does. This can result in missed opportunities for early intervention and potential health consequences for the affected individual ( Golbus et al., 1979).
CHALLENGES OF PRENATAL SCREENING
Trisomy screening presents several challenges that must be taken into account. These challenges include the following:
Gestational age: The optimal time for trisomy screening is during the first trimester of pregnancy when the risk of certain genetic abnormalities is highest. However, some screening tests may not be accurate at this early stage ( Avgidou et al., 2005).
Individual variation: Every pregnancy is unique, and the risk of certain genetic abnormalities can vary depending on factors such as family history and ethnicity. Individual variations can make it difficult to determine an accurate screening result ( Dondorp et al., 2015).
Noninvasiveness: The less invasive nature of certain screening tests, such as ultrasound, is appealing to many pregnant women. However, it is important to note that ultrasound screening alone is not a reliable method for diagnosing trisomy ( Allyse et al., 2015).
Psychological impact: Trisomy screening can have a significant psychological impact on both pregnant women and their partners. The uncertainty surrounding the results of a screening test can be overwhelming ( Bedei et al., 2021).
In brief, trisomy screening is a powerful tool for detecting certain genetic abnormalities during pregnancy. However, it is important to note that the accuracy and reliability of the tests can vary depending on various factors. Pregnant women should discuss the risks and benefits of trisomy screening with their healthcare providers to make an informed decision about their own healthcare needs.
FUTURE DIRECTIONS
Prenatal screening is a critical aspect of prenatal care that aims to identify potential genetic disorders early in pregnancy. Traditionally, serum screening and amniocentesis have been the primary methods used to detect T21 and other trisomies. However, with advancements in molecular biology, novel biomarkers have been identified that have the potential to be added to existing prenatal screening programs ( Ilekis et al., 2016).
Identification of novel biomarkers
The identification of novel biomarkers has revolutionized the field of prenatal screening and has opened up new possibilities for the early detection of genetic disorders ( Pös et al., 2019). These biomarkers, which are measurable substances in maternal serum or amniotic fluid, can provide valuable information about the fetus’ health status. By incorporating these biomarkers into existing screening programs, healthcare providers can enhance the specificity and sensitivity of screening, leading to improved outcomes and reduced healthcare costs ( National Academies of Sciences Engineering, and Medicine, 2016).
Integration into existing prenatal screening programs
The integration of novel biomarkers into existing prenatal screening programs requires careful consideration and collaboration among various stakeholders, including healthcare professionals, researchers, and policymakers ( Monaghan et al., 2016). One potential approach is to integrate these biomarkers into existing serum screening assays, increasing diagnostic accuracy and reducing the number of false positives or false negatives compared to traditional screening methods. Additionally, novel biomarkers could also be used as a standalone screening method or combined with existing screening modalities, such as ultrasound or amniocentesis ( Bahtiyar et al., 2007).
Impact on healthcare practices
The integration of novel biomarkers into existing prenatal screening programs has the potential to revolutionize healthcare practices. By providing more accurate and reliable screening results, healthcare providers can make more informed decisions regarding the management of pregnancies. This could include offering additional diagnostic tests or interventions to women who have a higher risk of developing certain genetic disorders. Additionally, the availability of novel biomarkers could lead to earlier diagnosis, allowing appropriate interventions and counseling for families affected by genetic disorders ( Mamas et al., 2011).
Ethical considerations
The integration of novel biomarkers into existing prenatal screening programs raises important ethical considerations. These considerations include the potential for increased invasiveness, the potential to influence reproductive decision-making, and the need to balance the benefits and limitations of additional testing. It is crucial to establish clear guidelines, informed consent processes, and mechanisms to ensure informed choice and autonomy for women considering prenatal screening ( Laufer-Ukeles, 2011).
TECHNOLOGICAL ADVANCEMENTS, ANTICIPATION OF FUTURE TECHNOLOGICAL ADVANCEMENTS, AND THEIR POTENTIAL INFLUENCE ON THE DEVELOPMENT OF ROBUST BIOMARKER-BASED SCREENING METHODS FOR T21 AND T18
Technological advancements have revolutionized various industries, including healthcare. In the field of prenatal screening, technological advancements have the potential to significantly impact the development of robust biomarker-based screening methods for T21 and T18. Here, we explore some of the anticipated future technological advancements and their anticipated influence on these screening methods.
Artificial intelligence and machine learning
Artificial intelligence (AI) and machine learning (ML) have shown great potential in analyzing complex medical data ( Ahmed et al., 2020). These technologies can analyze vast amounts of genetic and clinical information to identify patterns and predict disease risk ( Fig. 2). By integrating AI and ML into biomarker-based screening methods, scientists can develop more accurate and sensitive algorithms that can flag potential cases of T21 and T18 at an early stage ( Alsuliman et al., 2020).
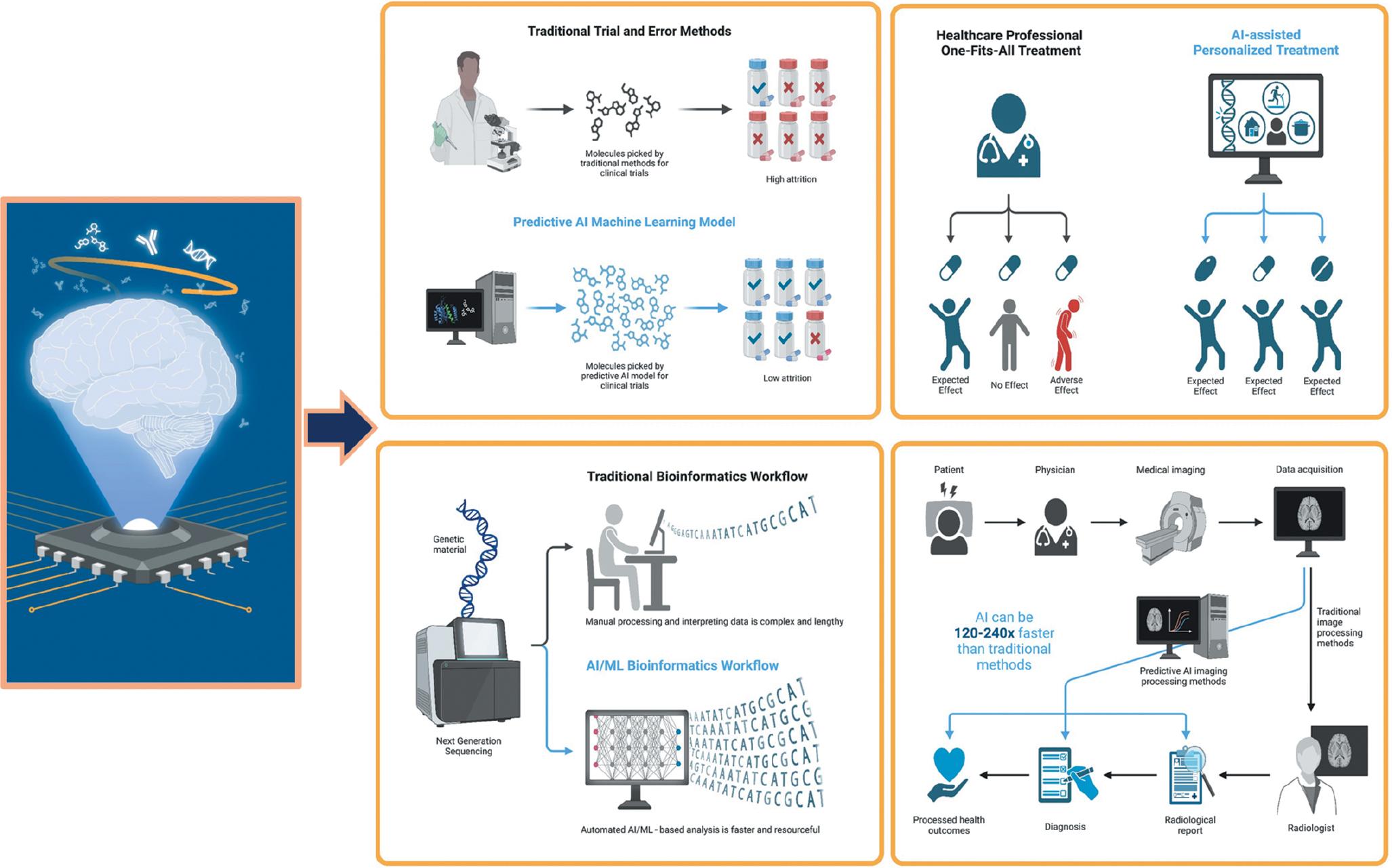
Utilizing the potential of AI in medicine to improve patient care. AI can help automate processes such as diagnosis, testing, and treatment planning, leading to better accuracy and faster treatment times. AI can also be used to provide personalized care to patients, as AI-based systems can analyze patient data in real time and adjust treatments accordingly. Abbreviations: AI, artificial intelligence; ML, machine learning.
Wearable devices and telemedicine
Wearable devices and telemedicine technologies offer immense potential in remote health monitoring and prenatal care. These devices can collect various physiological data from pregnant women, including heart rate, blood pressure, and fetal movements. Analyzing these data combined with genetic and clinical data can help identify early signs of T21 and T18, enabling timely interventions.
Nanoparticles and targeted delivery
Nanoparticles offer unique properties that make them suitable for drug delivery and targeted therapies ( Fig. 3). These tiny particles can be engineered to carry specific therapeutic agents or diagnostic molecules, allowing for improved accuracy and precision in biomarker-based screening methods ( Yetisgin et al., 2020). By incorporating nanoparticles into screening assays, scientists can enhance the detection and counting of specific biomarkers associated with T21 and T18.
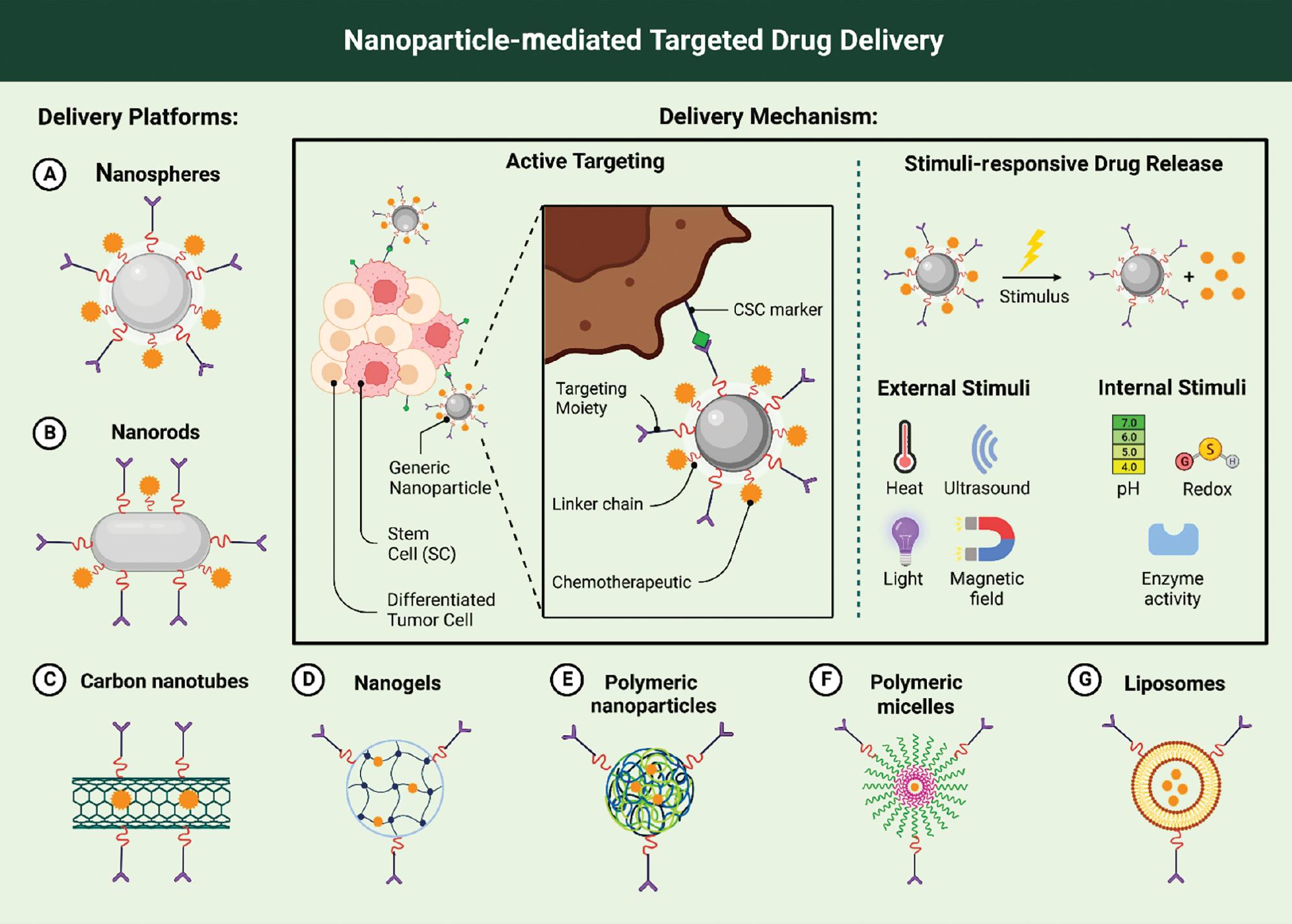
The use of nanoparticles and targeted delivery systems. The use of nanotechnology enables much smaller particles to be produced than those used in conventional pharmaceuticals, allowing them to penetrate cells and tissues more easily than other drug molecules. Using nanoparticles in conjunction with targeted delivery systems allows doctors to precisely target specific parts of the body, delivering drugs at specific locations within the body with pinpoint accuracy.
Genetic editing and gene therapy
Genetic editing and gene therapy have emerged as promising approaches for treating genetic disorders. These technologies involve altering or correcting faulty genes, offering a potential cure for T21 and T18 ( Doudna, 2020). While genetic editing is not yet feasible for prenatal screening, it may hold promise for future advancements. Gene therapy, on the other hand, may enable targeted delivery of corrective genes to the fetus, offering a potential cure for T21 and T18 ( Chen et al., 2022). In brief, regarding technological advancement, it holds immense potential for the development of robust biomarker-based screening methods for T21 and T18. Genetic sequencing and analysis, AI and ML, wearable devices and telemedicine, nanoparticles and targeted delivery, and genetic editing and gene therapy are all areas that show promise in improving the accuracy, sensitivity, and efficiency of prenatal screening. As these technologies continue to evolve, they will undoubtedly revolutionize the field of prenatal screening, leading to better detection, diagnosis, and interventions for T21 and T18.
CONCLUSION
This review underscores the continuous endeavors in prenatal screening to uncover biomarkers for T18 and T21 pregnancies. It stresses the importance of ongoing research, collaboration, and technological advancements to refine the accuracy and accessibility of screening methods. While traditional biomarkers like maternal serum biochemistry and ultrasound have been pivotal in trisomy screening, they have limitations in sensitivity and specificity. Recent focus has shifted to emerging biomarkers, notably fetal DNA analysis, offering advantages like noninvasiveness and potential early detection. However, further refinement is necessary to enhance accuracy and cost-effectiveness. Exploration into placental and maternal miRNAs, among other markers, presents promising avenues for early trisomy detection. Yet, validation and standardization are imperative. Collaboration across disciplines is crucial to advance screening protocols. Technological innovation, including user-friendly devices and cloud-based platforms, can democratize access to screening and real-time monitoring, benefiting both individuals and at-risk pregnancies. A balanced approach, integrating traditional and emerging technologies, optimizes screening. Traditional markers offer crucial insights into gestational age and fetal well-being, while emerging biomarkers present potential for earlier detection and improved specificity.