1. INTRODUCTION
Depression, a central nervous system (CNS) disorder, has a high prevalence and is associated with personal and socioeconomic costs [1, 2]. Despite the immense network of the CNS, >98% of small-molecule drugs and nearly all large-molecule drugs administered peripherally are unable to cross the blood-brain barrier (BBB) [3, 4]. The BBB prevents most foreign substances, even those with therapeutic benefit, from entering the brain via the circulation [5].
Nose-to-brain delivery, which bypasses the BBB to deliver molecules directly to the brain, is a potentially effective alternative to invasive drug delivery methods [6, 7]. Nose-to-brain delivery avoids the enzymatic and microbial drug degradation in the gastrointestinal tract and first-pass impact that can occur in the liver when pharmaceuticals are administered systemically [8–10], all of which facilitate increased brain bioavailability and decreased peripherally induced adverse effects [11]. Compliance with intranasal administration is good and is convenient for the patient [12]. The nose-to-brain pathway is mainly divided into the olfactory and trigeminal pathways [13, 14]. Olfactory receptor neurons are interspersed among supporting and basal cells to form the olfactory epithelium [15, 16]. The drug traverses the neuron axon in the olfactory pathway, passes the bundle of nerves extending over the sieve plate, and ultimately enters the olfactory bulb situated on the cerebral surface. The olfactory bulb is the only peripheral region of the body that maintains direct contact with the CNS [17]. Trans-epithelial penetration of drugs through the olfactory epithelium also contributes to drug delivery to the brain after intranasal administration via the cerebrospinal fluid (CSF) [18].
Nanoparticles are sub-particulate drug delivery systems prepared by adsorbing or encapsulating a drug in a carrier material [19]. Micelles are commonly used carriers that have the ability to improve brain targeting by enhancing drug solubility [20], which extends the duration of drug retention [21] and increases the drug concentration in brain tissue through nose-to-brain delivery [22]. Solutol HS15 is widely used as a nasal mucosal absorption enhancer [23]. The mechanism of action for Solutol HS15 primarily involves the combined effect on the cell membrane and the effect on actin organization in the cytoskeleton, which opens tight junctions and enhances the absorption of drugs through the mucosa [24]. Additionally, Solutol HS15, a solubilizer approved by the Food and Drug Administration (FDA) for injectable drug delivery, is frequently used to overcome the issue of intravascular administration of insoluble pharmaceuticals [25]. Based on the above considerations, Solutol HS15-based nanoparticles will be designed in this study to achieve nose-to-brain targeted delivery of agomelatine (AGO).
AGO was chosen as a model drug for the current study. AGO is a relatively new antidepressant with a mechanism of action that differs from other antidepressants [26]. AGO has structural similarities to melatonin (MLT), functioning as an agonist. AGO has comparable affinity to MLT for binding to MLT1 and MLT2 receptors [27]. Moreover, AGO antagonizes serotonin 5-HT2C receptors, whereas the neuronal uptake of monoamines is not affected [28]. A micelle system was developed based on the excellent properties of Solutol HS15 wherein the micelles were utilized for encapsulation of AGO. We aimed to determine whether nose-to-brain drug delivery combined with Solutol HS15 represents a useful drug delivery system for increasing drug bioavailability in the CNS.
2. MATERIALS AND METHODS
2.1 Materials
AGO (98% pure) and cyanine7 amine were purchased from Aladdin Chemical Inc. (Shanghai, China). Solutol HS15 was kindly supplied by BASF Co., Ltd. (Shanghai, China). Antifade mounting medium with DAPI was obtained from Dalian Meilun Biotech Co., Ltd. (Liaoning, China). Tissue-Tek®O.C.T. compound was purchased from Sakura Global Holding Co., Ltd. (Hong Kong, China). Phosphate-buffered saline (PBS) was obtained from Wuhan Servicebio Technology Co., Ltd. (Wuhan, China). Absolute ethanol was acquired from Sigma-Aldrich (Alexandria, UK). Amicon® Ultra-4 mL Centrifugal Filter Units (3 KDa MWCO) was purchased from Merck Millipore (Billerica, MA, USA). All other reagents were purchased from Shan Dong Yu Wang Reagent Co. (Shandong, China).
2.2 Animals
Healthy female Sprague-Dawley rats (6–8 weeks old) were purchased from Liaoning Changsheng Biotechnology Co., Ltd. (Liaoning, China) and housed in a pathogen-free, light-cycled, temperature-controlled facility. All animal experiments were performed according to the guidelines approved by the Institutional Animal Use Committee of Shenyang Pharmaceutical University and the National Research Council’s Guide for the Care and Use of Laboratory Animals.
2.3 Preparation of HS15-AGO
AGO-loaded micelles (HS15-AGO) were prepared using the rotary evaporation method. AGO and Solutol HS15 were dissolved in solvent ethanol. The solution was stirred at room temperature for 5 min and the solvent was allowed to evaporate at 40°C under vacuum in a rotary evaporator (RE-5299; YuHua, Shanghai, China). The thin film formed was hydrated with 2 mL of PBS and the mixture was stirred vigorously at 37°C with continuous rotation until micelles containing AGO were formed. Dialysis against PBS was performed in a dialysis bag (molecular weight cut-off [MWCO] = 3.5 kDa) to remove unentrapped drug. The purified dispersion was filtered through a 0.45-μm microporous filter to remove microparticulate impurities and the filtrate contained pure AGO-loaded micelles.
2.4 Preparation of HS15-Cy7
Cyanine7 amine-loaded micelles (HS15-Cy7) were prepared using the rotary evaporation method ( Scheme 1a ). Cy7 and Solutol HS15 were dissolved in solvent ethanol. The solution was stirred at room temperature for 5 min and the solvent was allowed to evaporate at 40°C under vacuum in a rotary evaporator. The thin film formed was hydrated with 200 μL of PBS and the mixture was stirred vigorously at 37°C with continuous rotation until the micelles containing AGO were formed. Ultracentrifuge tubes were used to remove the Cy7 that was not loaded. The liquid was applied to Amicon® Ultra-4 ml centrifugal filters (MWCO = 3.5 kDa MWCO) and spun at 4000 × g for 30 min (HSC-3020L; SCIENTZ, Zhejiang, China). The concentrate was collected from the sample reservoir of the filtration unit using a pipette tot. PBS was added to the concentrate and washed until the filtrate was colorless. The purified dispersion from the sample reservoir of the filtration was filtered through a 0.45-μm microporous filter to remove microparticulate impurities and the filtrate contained Cy7 amine-loaded micelles.
2.5 Characterization
The particle size distribution, polydispersity index (PDI), and ζ-potentials of micelles were measured by dynamic light scattering [DLS] (Nano-ZS90 nanosizer, Malvern Instruments, Malvern, UK). The HS15-AGO morphology and mesoporous nanostructure were observed by transmission electron microscopy [TEM] (Tecnai G2 F30; FEI, Eindhoven, The Netherlands).
2.6 Determination of AGO encapsulation efficiency
The pure AGO-loaded micelles were fixed to 2 ml with ethanol, followed by ultrasonication at 400 W for 15 min to extract AGO from the core of the micelles. The resulting solutions were filtered by passing through a 0.45-μm membrane filter and estimation was carried out at 303 nm on a UV-visible spectrophotometer (UV-2150; UNICO, Dayton, NJ, USA). The drug loading (DL%) and drug encapsulation efficiency (DEE%) were calculated with the following formulas following equations (1) and (2), respectively.
Where W encapsulated, W total, and W micelles represent the weight of AGO encapsulated in the micelles, the initial AGO total weight, and the weight of AGO-loaded micelles, respectively.
2.7 In vitro drug release behaviors
In vitro drug release profiles were studied using the dialysis method [29]. A sample containing 4 mg of drug was loaded into a dialysis bag (MWCO = 3.5 kDa) and dialyzed against 10 mL of nasal fluid (pH 6.8) or CSF (pH 7.2) in a thermostatic oscillator at 37°C (200 rpm). AGO solution was used the control. DSMO (1% w/v) was added to the release medium to facilitate solubility [4]. At each predetermined timepoint, 2 mL of AGO solution was withdrawn and replaced with fresh dialysis media to maintain sink conditions. The sample solution was determined by a UV detector at 303 nm. The AGO solution was dialyzed as a 100% release control to eliminate non-specific adsorption of the drug to the dialysis membrane [4].
2.8 Stability of HS15 micelles
Micelles were made and stored in the liquid state at 4°C for 14 d. The PDI data and changes in the particle size distribution were examined as a function of time. In addition, the stability of the micelles was investigated by diluting the micelle solution with CSF and PBS solution, and the particle sizes of micelles were measured after 1, 2, 6, 10, 12, 14, and 24 h.
The influence of the administration technique on particle size was evaluated and the prepared HS15-AGO formulation was introduced into a nasal spray device. The variations in particle size, observed before and after passage through the nasal spray mechanism, were thoroughly assessed.
2.9 Optical imaging of HS15 micelles in rats
HS15-Cy7/Cy7 solution (100 μL) was administered to SD rats (n=3) by intranasal administration. Rats were initially scanned at 1, 2, and 4 h, after which 4 h was chosen to perform ex vivo imaging. All images were analyzed using Living Image® 4.3.1 software (Caliper Life Sciences, Hopkinton, MA, USA). The animals were anesthetized with a with a mixture of 1.5% isoflurane and 98.5% oxygen to sustain sedation throughout the imaging protocol. The brain and other major organs (heart, liver, lungs, spleen, and kidneys) were rapidly excised. Ex vivo imaging was carried out immediately. The cryosectioning technique was been employed to prepare brain tissue samples and stained the nuclei with DAPI, which fluoresces blue under microscopic observation. Subsequently, we conducted detailed scans of these sections using a confocal microscope.
2.10 Pharmacokinetics studies
Three rats were fasted for 12 h with free access to water before administration for the pharmacokinetic studies. The CSF and plasma samples were collected after administration of HS15-AGO at 0.25, 0.5, 1, 3, and 6 h. The AGO content was determined by HPLC-MS/MS (TQ6500; SCIEX, Framingham, USA). Pharmacokinetic parameters were derived from drugs concentration-time data.
2.11 In vivo biodistribution studies
The Franz diffusion cell was used for in vivo biodistribution studies. Physiologic saline (14 mL) was added into the receiving chamber and the air bubbles were discharged. The sheep nasal mucosa layer was fixed between the receiving and supplying chambers, placed on a thermostatic water bath at 37°C, and equilibrated for 30 min. HS15-Cy7 formulation (200 μL) was administered to the nasal mucosa within the supply chamber. Following a 4-h incubation, the nasal mucosa was excised, rinsed, and fixed in 4% paraformaldehyde for 24 h. The segments were frozen in cryoembedding media, sectioned at a thickness of 10 μm, and placed on glass slides. The section results were observed by confocal microscopy (C2+; Nikon, Tokyo, Japan).
2.12 Histopathologic studies
After administration of HS15-AGO, the rat nasal mucosa, hearts, livers, spleens, lungs, and kidneys were isolated. Nasal mucosa treated with isopropanol and PBS (pH 7.4) was used as a positive and negative control, respectively. All the sections were stained with hematoxylin and eosin and fixed on the slides. The slides were observed under a light microscope.
3. RESULTS AND DISCUSSION
3.1 Characterization of HS15 micelles
HS15-AGO was characterized based on the hydrodynamic diameter, PDI, and zeta potential. HS15-AGO exhibited a diameter of approximately 14.17 ± 0.72 nm using dynamic light scattering ( Figure 1b ). A schematic diagram of HS15-AGO micelles is shown in Figure 1a . Further investigation into the size and morphology of HS15-AGO via TEM revealed the micelle shapes in Figure 1c . The micelle structures appeared spherical or near-spherical with a uniform distribution in size and no evidence of aggregation. This ultra-small micelle size corroborated the nanocluster dimensions observed through dynamic light scattering. In addition, the particle size was uniformly dispersed with a PDI coefficient of 0.081 ± 0.023. HS15-AGO had a zeta electric potential of approximately −2.32 ± 0.44 mV.
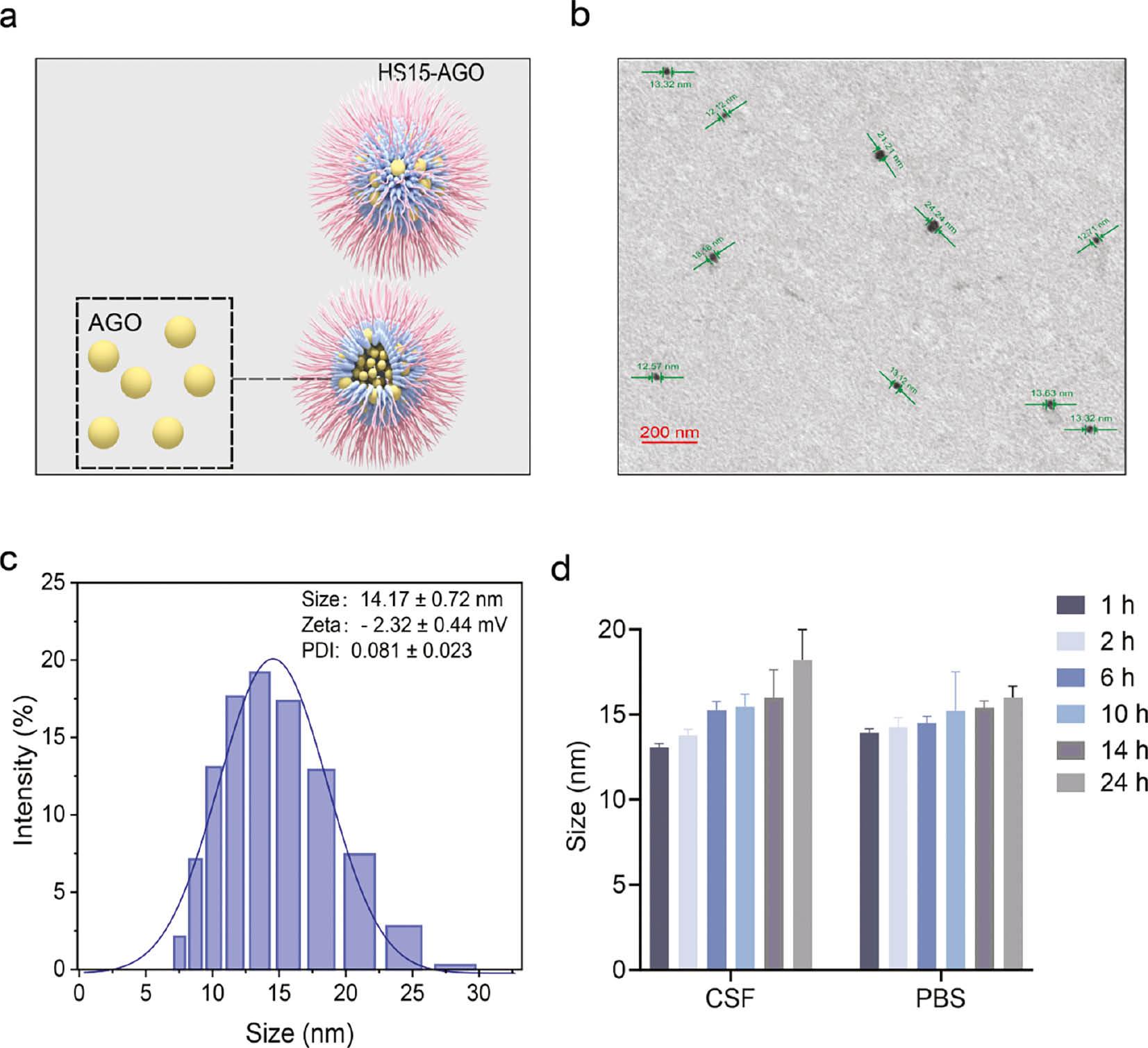
Diagram showing the characterization of micelles.
(a) Schematic diagram of HS15-AGO micelles. (b) TEM images of HS15 micelles [scale bar, 200 nm]. (c) Particle size of HS15 micelles. Data are the mean ± SD (n=3). (d) The particle sizes of the micelle solution diluted with the CSF and PBS solution after 1, 2, 6, 10, 12, 14, and 24 h.
HS15, a non-ionic surfactant, typically yields micelles with zeta potentials close to zero due to the uncharged, polar head groups, which impart a minimal electrostatic charge to the micelle surfaces. For HS15-AGO micelles, the stability is predominantly governed by steric hindrance. HS15 contributes to forming an adsorption layer around each particle, creating a physical barrier that prevents particle aggregation. This steric stabilization mechanism, involving spatial hindrance and the thickness of the layer, ensures dispersion stability, even when the zeta potential is low or near zero.
The particle sizes of HS15-AGO in CSF and PBS solutions are summarized in Figure 1d and Table S1 , respectively. The stability of the HS15-AGO micelles was evidenced by the unchanged sizes after dilution with PBS over 24 h, suggesting that these micelles preserves stability within the delivery device before administration. Similarly, the consistent size of HS15-AGO post-dilution with CSF indicated the capacity to retain micellar morphology within CSF, then achieved slow release, further affirming the suitability for effective drug delivery. The above results suggested that our constructed system of HS15 micelles existed in an intact form before and after intranasal administration, therefore HS15 micelles were further used for subsequent studies.
3.2 DL% and DEE%
The DL% and DEE% of the formulations was carried out using UV spectrophotometry. The DL% and DEE% data for prepared HS15 micelles were 96.96% and 4.41%, respectively. The notably high encapsulation efficiency of AGO within the HS15-AGO suggested that HS15 micelles were primed for subsequent exploration as an innovative nose-to-brain drug delivery system.
3.3 In vitro release studies
The release of AGO from HS15 micelles was studied up to 10 h in 10 mL of nasal fluid and CSF ( Figure 2a ). The in vitro release data are listed in Tables S2 and S3 . The AGO solution can be released 100% in 2h. HS15-AGO can be released more slowly in CSF and a 100% release was achieved after 4 h in the CSF. AGO achieves slow release in HS15 micelles. This suggested that the preparation of AGO into micelles had a sustained-release effect on AGO.
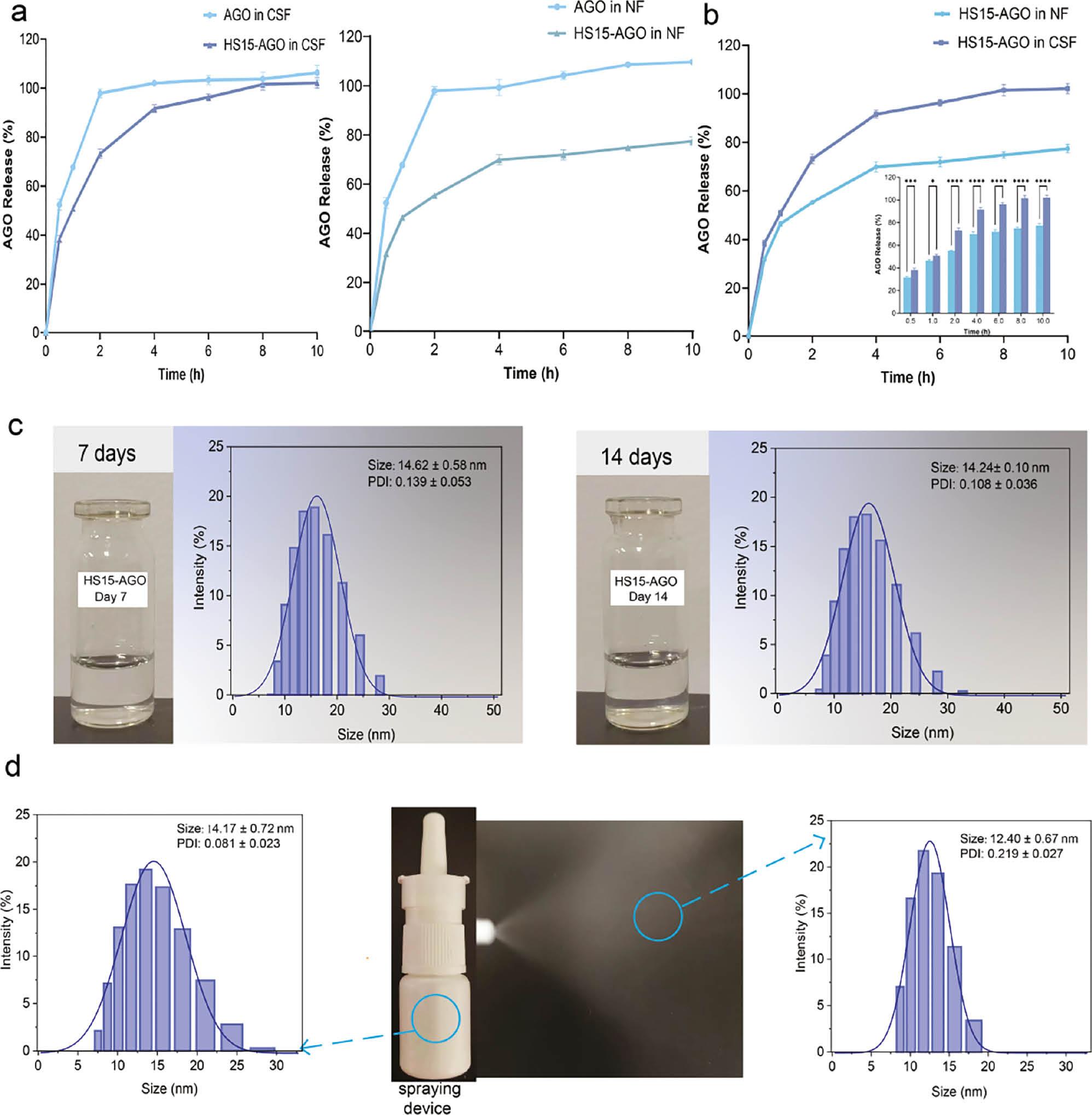
Diagram showing stability and in vitro release behavior of HS15-AGO.
(a) The release of AGO from HS15 micelles in nasal fluid or cerebrospinal fluid. (b) Comparison of cumulative release of HS15-AGO in nasal fluid and cerebrospinal fluid. (c) The appearances and particle sizes of HS15-AGO stored at 4°C for 7 and 14 d. (d) The state of HS15 polymer micelles when ejected and the changes in particle sizes before and after ejected. All experiments were repeated two-to-three times.
In addition, an approximate 80% release of AGO over 4 h was achieved, a value that was not altered significantly by the time changes in the NF. A slightly higher drug release was achieved with HS15-AGO in the CSF after 10 h. This finding might be because the cumulative release in the nasal fluid was small due to the pH ( Figure 2b ). The results showed that the environment of the CSF is favorable to the release of AGO from HS15 micelles.
It was easily shown that the preparation of HS15 into micelles possessed a sustained drug release behavior and the ability to release the full amount of drug in the CSF. There were several factors that impacted the length of time a drug could be effective, among which clearance in the brain was one of the most important aspects that needed to be considered, in addition to the time of drug release from the carrier. The stability of the HS15-AGO micelles was evidenced by the unchanged sizes after dilution with PBS over 24 h, suggesting that these micelles preserved stability within the delivery device before administration [30]. Similarly, the consistent size of HS15-AGO post-dilution with CSF indicated the capacity to retain micellar morphology within the CSF, then achieved slow release, further affirming the suitability for effective drug delivery [31]. The above results suggested that the constructed HS15 micelle system existed in an intact form before and after intranasal administration. Therefore, HS15 micelles could be further used for subsequent studies.
3.4 Stability of HS15 micelles
After the micelles were stored at 4°C for 7 and 14 d, no apparent change in color and appearance was observed ( Figure 2c ). The particle sizes were essentially constant and the PDI remained favorable. These characteristics exhibited minimal variance, suggesting that the HS15-AGO micelle solution remained stable at 4°C.
The change in particle size before and after spraying was measured. The results indicated that the micelles before and after spraying were uniformly spherical, with average diameters of 14.17 ± 0.72 and 12.40 ± 0.67 nm, respectively ( Figure 2d ). The particles remained well dispersed. The results demonstrated that the shear force of the drug delivery device had less effect on the micelles and the micelles were stable.
3.5 Brain biodistribution studies by in vivo and ex vivo optical imaging in rats
HS15-Cy7 micelles were used to study the brain distribution in vivo and in vitro. Cy7 solution was used as the control group. Considering the maximum dosages acceptable for intranasal administration in rats, the HS15-Cy7 and Cy7 solution (100 μL) were administered to SD rats by intranasal administration. The location of brain tissue in the rats is shown in Figure 3a . Representative whole-body images at 1, 2, and 4 h and ex vivo images of brain tissues harvested at 4 h ( Figure 3b, 3c ), signals in brain tissues were semi-quantitatively analyzed ( Figure 3d, 3e ). All images were obtained using the Cy7 filter (740/760 nm for excitation/emission wavelengths) with an exposure time of 1 s.
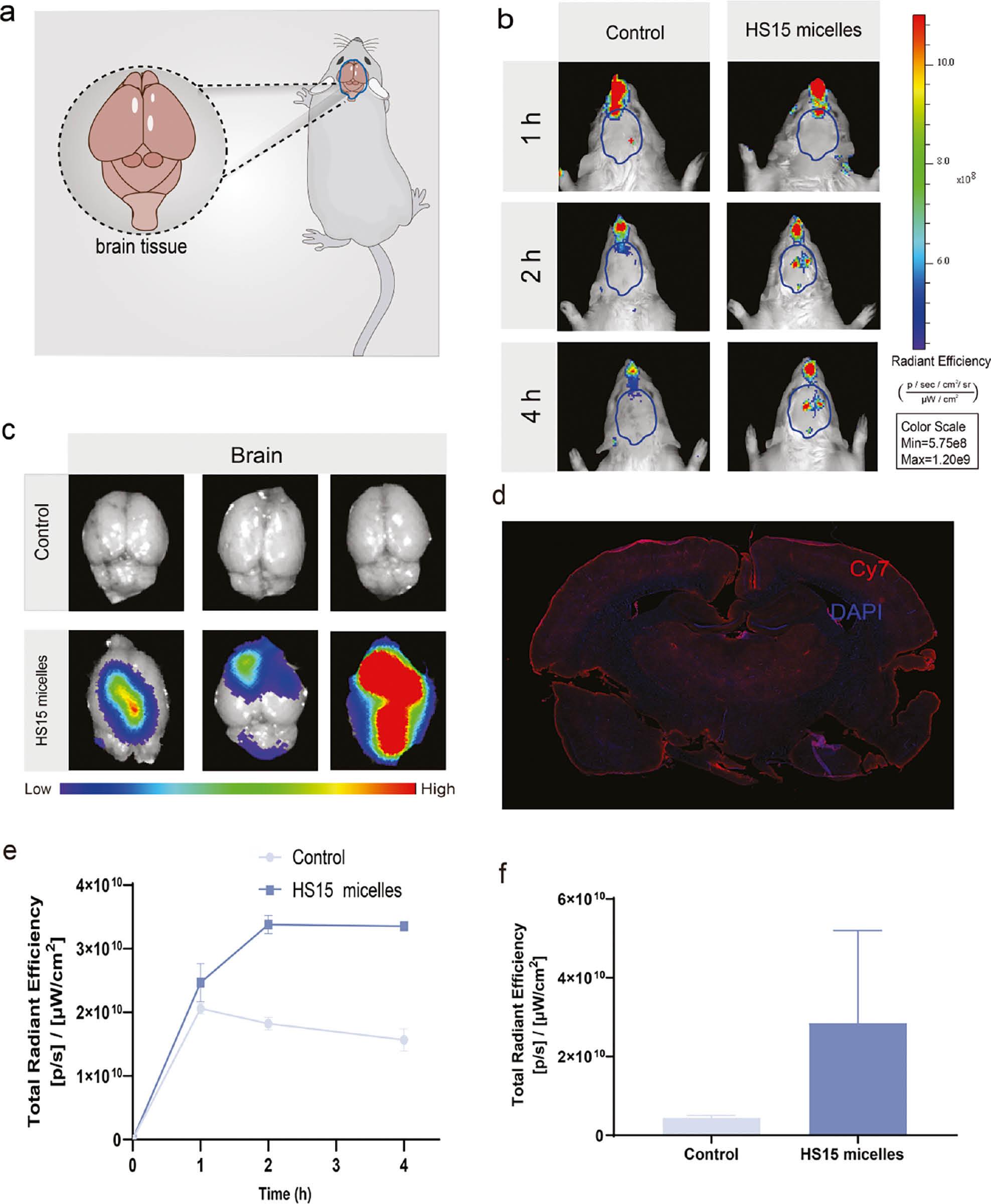
Brain biodistribution in SD rats after intranasal administration.
(a) Location of brain tissue in the rats. (b) Representative body in vivo images at 1, 2, or 4 h after intranasal administration. (c) Ex vivo quantification of fluorescence signals of Cy7 and HS15 micelles of brain tissue at 4 h. (d) Images of coronal section. (e) In vivo quantification of fluorescence signals of mice at 1, 2, and 4 h. (f) Total radiant efficiency of brain tissue 4 h after injection. All experiments were repeated two-to-three times.
Fluorescence signals were detected in the brain in both groups 1 h after intranasal administration of although the control group was lower. The maximum intracerebral fluorescence intensity was reached at 1 h in the control group; the intracerebral fluorescence intensity gradually decreased thereafter. This finding might be because Cy7, as a small molecule, entered the brain tissue in small amounts through the olfactory epithelium after intranasal administration and was metabolized quickly. In contrast, the fluorescence intensity in the HS15 micelle group continued to increase after 1 h and reached the peak value at 2 h. The fluorescence intensity slightly decreased but was still higher than the control group. In addition, ex vivo images of HS15-Cy7 were administered via intranasal administration and exhibited significantly higher fluorescence intensity in the brain than the control group, also demonstrating the ability of HS15 micelles to reach the brain tissues.
After 4 h of intranasal administration, the brain tissue was removed and a significant fluorescence of the HS15 micelles compared to the control group was observed ( Figure 3c, 3f ). To confirm the penetration of the drug into the brain parenchyma, brain tissue from the HS15-Cy7 treated group underwent cryosectioning. Nuclei stained with DAPI dye exhibited blue fluorescence under confocal microscopy. Cy7 was used for near-infrared emission and rendered as red in our images. This process ascertained that the vector facilitated drug entry into critical areas of the brain parenchyma, including regions like the hippocampus and amygdala ( Figure 3d ).
3.6 Pharmacokinetics
DTE% is a measure of the brain targeting of drugs [32]. After intranasal administration, the DTE% was 76.66%. The results indicate that HS15-AGO is highly efficient in direct transmission via transnasal route. This result underscored highlighting the pronounced propensity for targeted brain accumulation. The peak concentration in the CSF was reached 0.25 h after administration, with AGO crossing the BBB and absorbed into the CSF 1 h after entering the bloodstream.
Drug delivery through the olfactory region is largely possible via the olfactory nerve as an intraneuronal pathway or the olfactory epithelial cells present around the nerve pathway. Transepithelial penetration of drugs through olfactory epithelial cells facilitates the delivery of drugs to the brain via the CSF after intranasal administration [33]. Previous studies reported that the kinetics of drug delivery via the intraneuronal pathway is markedly slower than that achieved through transepithelial transport via olfactory epithelial cells. Specifically, the temporal window for drug delivery through the intraneuronal route spans approximately 1.5–6 h post-administration, whereas transepithelial delivery can occur within minutes [17].
The fluorescence intensity of in vivo imaging combined with pharmacokinetic data showed that the drug entered CSF through the trans-epithelial permeation of olfactory epithelial cells reached the maximum in 15 min, and the drug was transmitted through the intraneuronal pathway within 2 h. In vivo imaging data reflected the common conditions of brain tissue and CSF ( Figure 3e ), while pharmacokinetic data reflected the drug conditions only in CSF ( Figure 4b ). This process was accompanied by a complex process in which the drug entered the cerebral tissues from the CSF and was expelled from the CSF. In this process the drug entered the brain tissue from the CSF and excreted from the CSF. Concurrently, incorporating the pharmacokinetic data observed in the bloodstream ( Figure 4a ), it was noted that a minor portion of the drug upon entering the bloodstream, penetrating the BBB, and reaching the brain approximately 1 h after administration. This process resulted in a pharmacokinetic profile characterized by a dual peak.
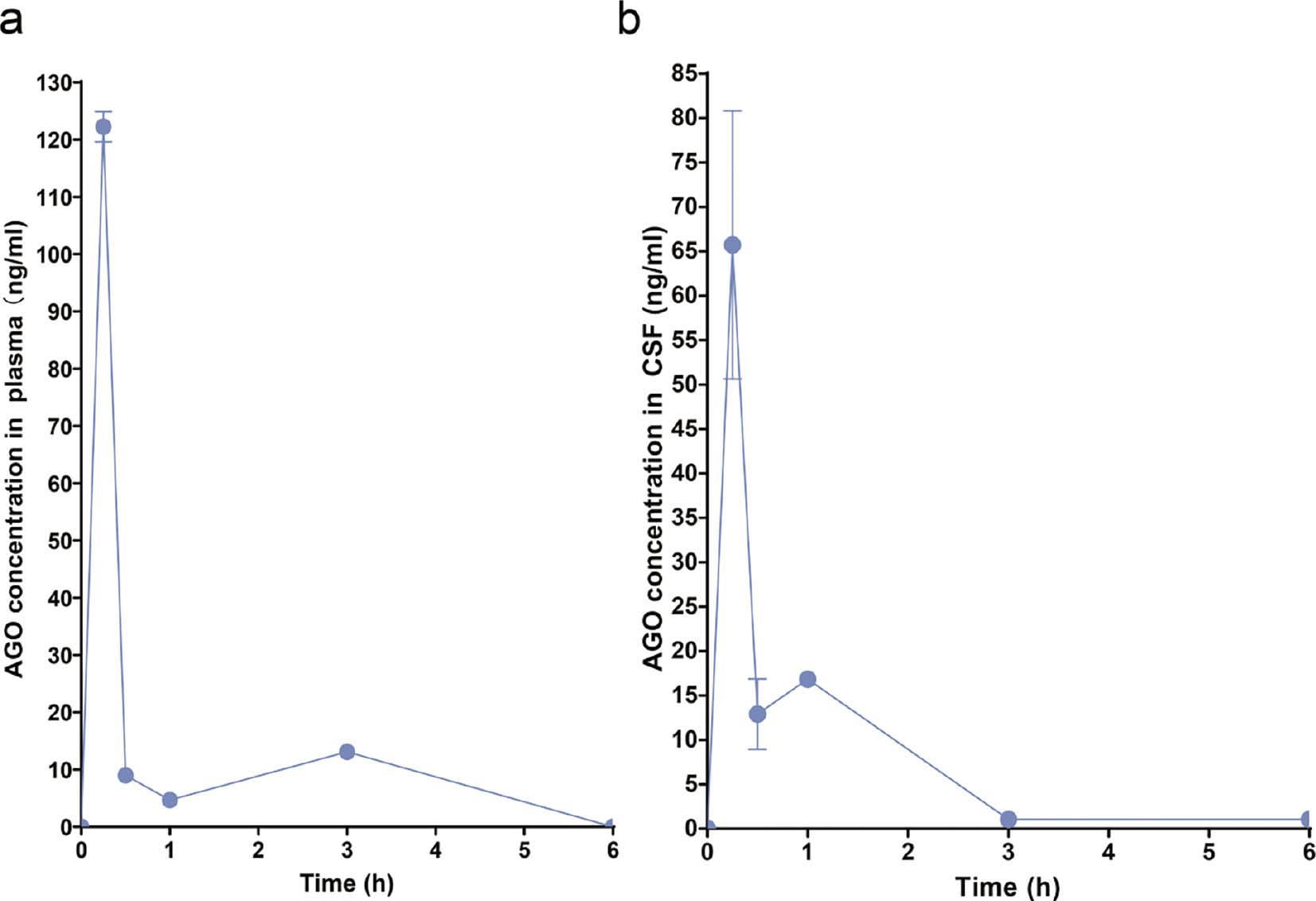
Pharmacokinetics studies of AGO.
(a) Plasma concentrations of AGO in rats after the administration of AGO at 0.25, 0.5, 1, 3, and 6h. (b) CSF concentrations of AGO in rats after the administration of AGO at 0.25, 0.5, 1, 3, and 6 h.
Overall, our results confirmed that encapsulating the drug in HS15 micelles combined with intranasal delivery could significantly help the drug to enter the brain tissue quickly and prolong the residence time in the brain compared to a pure drug solution.
3.7 Examination of nasal mucosal penetration of HS15 micelles
The cell nuclei in olfactory epithelial cells were stained with DAPI dye and showed blue fluorescence under a confocal microscope. Cy7 was utilized for its emission in the near-infrared range, which was visually represented as red in our images for illustrative purposes. As shown in Figure 5a and 5c , the fluorescent signal of HS15-Cy7 was observed in the olfactory epithelium. As shown in Figure 5b and 5d , DAPI and Cy7 were noted in the olfactory epithelium, which was shown by the fluorescence intensity of red and blue signals, indicating that there were large amounts of HS15 micelles that crossed the olfactory epithelium.
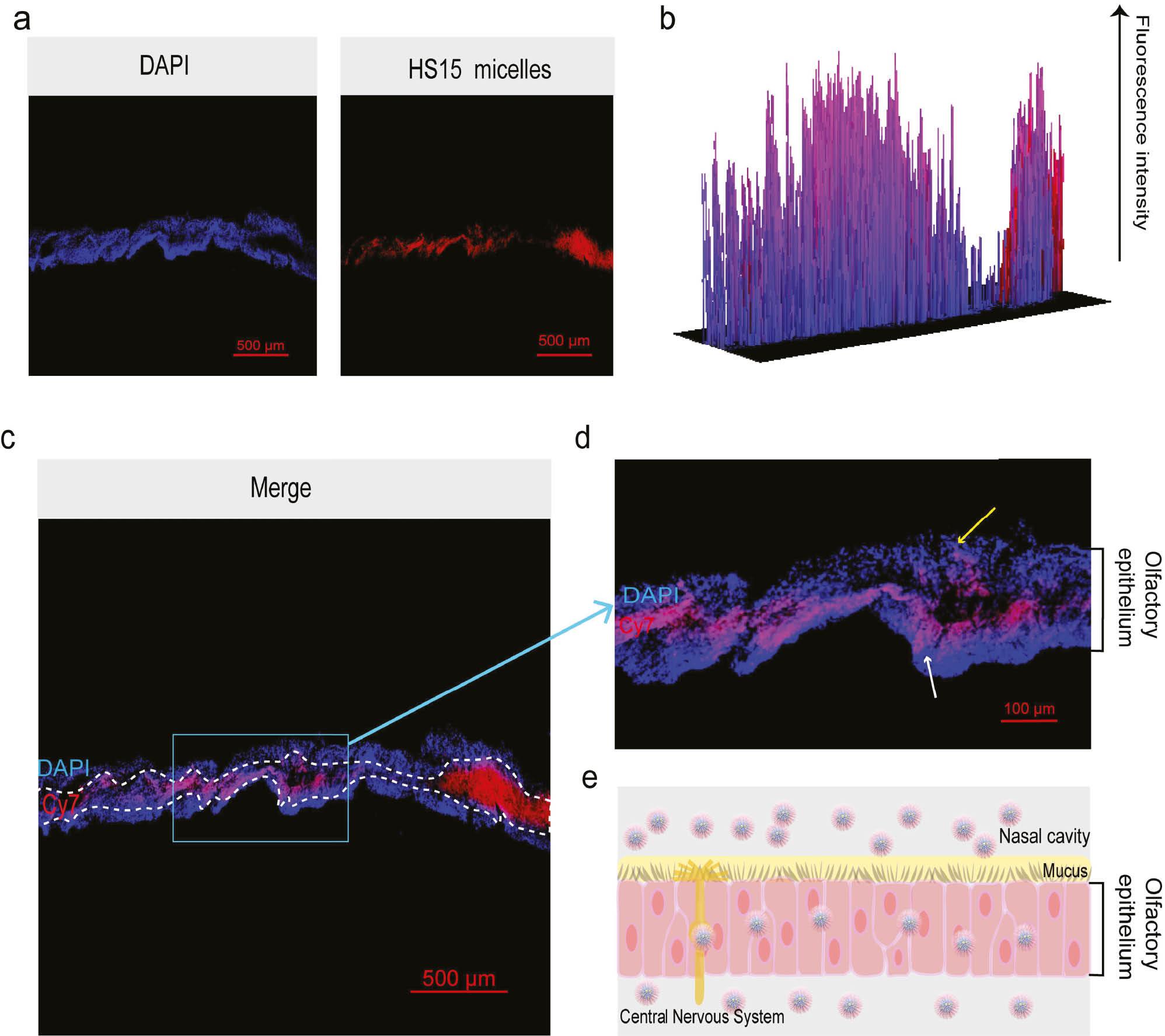
Diagram showing the transmembrane procedure of HS15 micelles and the change in structure during the transmembrane process.
(a) Confocal microscopic observation of DAPI and HS15 micelles. (b) Comparison of fluorescence intensity of DAPI and Cy7. (c) Confocal microscopic observation within the olfactory epithelium after emergence. (d) Locally enlarged image. (e) Schematic representation of the process of drug crossing the olfactory epithelium.
During the same process the red fluorescent signal emitted by Cy7 not only appeared on the surface of the olfactory epithelium, as shown by the yellow arrow, but also penetrated into the interior of the olfactory epithelium. Finally, red fluorescence passed through the olfactory epithelium where the white arrow pointed. This finding indicated that HS15 micelles assisted drugs to cross the olfactory epithelium into brain tissue ( Figure 5e ). Together these results suggested the accuracy of the experimental results of brain tissue in vitro and in vivo. HS15 micelles act as a carrier to assist drugs in crossing the olfactory epithelium into brain tissue.
Selvaraj et al. [34] reported that the drug passed along the olfactory nerves through the olfactory bulb via intra- and extra-neuronal transport into the olfactory epithelium, then delivered to the CNS (specifically, the cerebrum, cortex, and cerebellum). The administration of drugs through the olfactory area is mostly facilitated by the olfactory nerve as an intraneuronal pathway or the olfactory epithelial cells present around the nerve pathway [35]. Transcellular mechanisms, such as endocytosis and simple diffusion, as well as paracellular mechanisms facilitated by the junctions between cells, offer an additional means of drug transportation across olfactory epithelium cells [36]. With our experimental results, we thought that the micelle system we constructed used a pathway through the olfactory epithelium across the olfactory epithelium into the brain tissue.
3.8 Organ biodistribution studies by ex vivo optical imaging in rats
The organ influence of HS15 micelles was evaluated following intranasal administration. After 4 h of intranasal administration, the major organs was removed and photographed ( Figure 6a ). The signals in major organs were semi-quantitatively analyzed lower signals were observed in the hearts, lungs, spleens, livers, and kidneys with the HS15 micelles, and much higher signals were detected with the control group via intranasal administration. Notably, there were significant differences in the livers and lungs ( Figure 6b ).
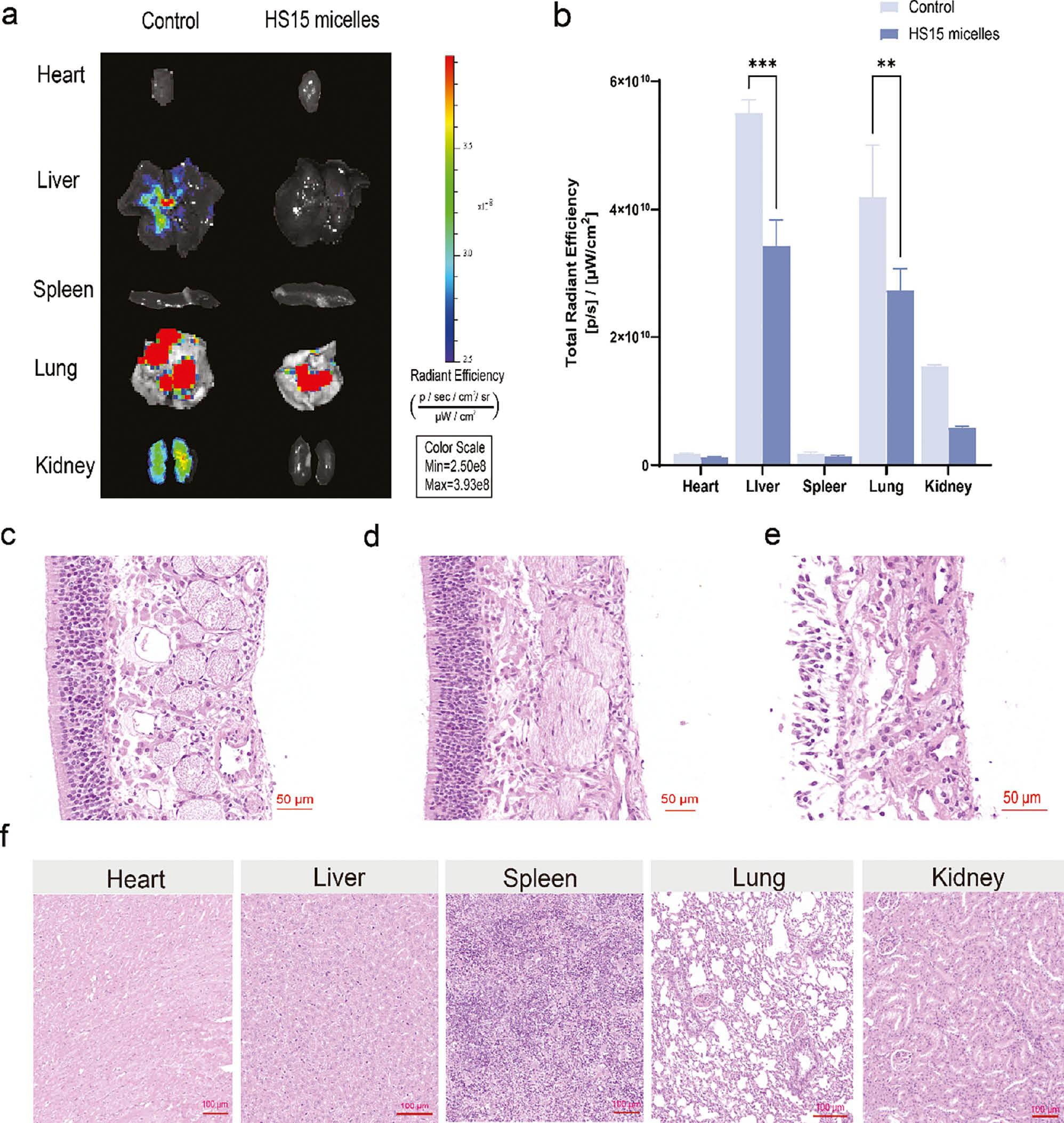
Ex vivo organ biodistribution in SD mice after intranasal administration and histopathologic study of HS15-AGO.
(a) Representative ex vivo images of excised organs 4 h after treatment. (b) Ex vivo quantification of fluorescence signals of Cy7 and HS15 micelles in excised organs at 4 h. Results are presented as the mean ± SD. **P<0.01, ***P<0.001. All experiments were repeated two-to-three times. (c) H&E staining of nasal mucosa: Negative control treated with saline. (d) H&E staining of nasal mucosa: Test treated with HS15-AGO. (e) H&E staining of nasal mucosa: Positive control treated with isopropyl alcohol. (f) H&E staining of major organs: Test treated with HS15-AGO.
The ex vivo imaging study confirmed that the intranasal administration offered a brain-targeting approach with low accumulation in the rest of the body. Mustafa et al. [37] prepared a homogenized chitosan-coated mucoadhesive ropinirole nanoemulsion that detected an insignificant ropinirole level in the systemic organs and detected significantly elevated levels in the brain. Similarly, these results suggested that insoluble formulation into HS15 micelles and administration intranasally increased the drug concentration in the brain and avoided non-specific liver accumulation. This result showed that HS15 micelles enhanced brain targeting and had the advantage of low systemic toxicity.
3.9 Histopathology studies
Rat nasal mucosa, hearts, livers, spleens, lungs, and kidneys were isolated after administration of HS15-AGO. Nasal mucosa treated with isopropanol and PBS (pH 7.4) was used as a positive and negative control, respectively. All the sections were stained by hematoxylin and eosin and fixed on the slides. The slides were observed under a light microscope. The nasal mucosa was isolated after administration of HS15 micelles. The saline and HS15-AGO groups showed that the epithelial parts were intact, with no inflammatory cell infiltration, hemorrhage, necrosis, or edema ( Figure 6c, 6d ), but the isopropyl alcohol group showed an incomplete epithelial part ( Figure 6e ). In addition, the cellular structure was intact and well-defined in the rat hearts, livers, spleens, lungs, and kidneys sections based on optical microscopy, and none of which showed obvious histopathologic abnormalities ( Figure 6f ). Together, these results suggest that our mode of administration and dosage did not result in systemic toxicity.
4. CONCLUSION
In this study we successfully constructed a HS15 micelle system to promote the drug across the olfactory epithelium through the olfactory-brain pathway to effectively target the brain tissue and improve the intracerebral bioavailability of the drug. The nanocarrier utilized HS15 with a surfactant function, which is highly safe, as the shell structure, and the drug was highly loaded within this shell structure. Our study demonstrated that HS15 micelles possess stable properties and resulted in lower accumulation in the rest of the body, suggesting the potential clinical value. Interestingly, HS15 micelles not only rapidly entered brain tissue but also prolonged the time for the drug to exert its efficacy, indicating the possibility of achieving sustained drug release with this carrier. In future corollary studies HS15 micelles could be explored as carriers for insoluble drugs targeting various CNS disorders through intranasal administration.